Innovations in Mechanical Computing: Kirigami-Inspired Systems Revolutionize Data Storage
Discover the groundbreaking advancement in mechanical computing inspired by kirigami. Researchers have developed a system using interconnected polymer cubes for data storage, bypassing traditional electronics and opening new possibilities in various fields.
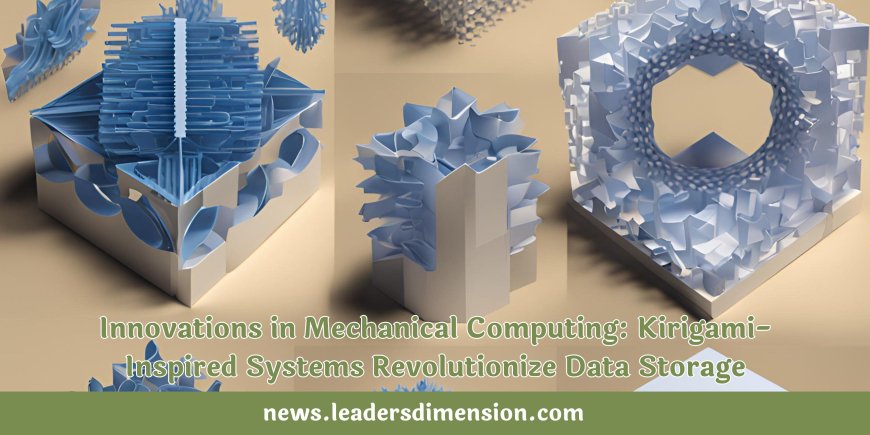
Mechanical computing has traditionally lagged behind its electronic counterpart, but recent developments inspired by the ancient art of kirigami may propel it into the forefront of technological innovation. Kirigami, a Japanese technique of paper cutting, has been creatively adapted by researchers to develop a new form of mechanical computer. This innovative system employs interconnected polymer cubes to store and process data, offering a promising alternative to conventional electronic computing.
The Kirigami Connection
Kirigami, similar to origami, involves cutting and folding paper to create intricate designs and structures. This method is not only aesthetically pleasing but also structurally robust. Researchers have drawn inspiration from these characteristics to create mechanical systems capable of complex computations.
In the context of mechanical computing, kirigami principles are applied to engineer materials that can change shape and configuration in response to specific stimuli. This flexibility is key to developing mechanical systems that can perform tasks traditionally managed by electronic components. By leveraging the adaptability and resilience of kirigami-inspired designs, scientists have paved the way for a new class of computing devices.
Polymer Cubes: The Building Blocks of a New System
At the heart of this innovative mechanical computer are interconnected polymer cubes. These cubes, made from specially designed materials, can change their configuration when subjected to external forces. Each cube acts as a basic unit of data storage, similar to bits in electronic computers.
The polymer cubes are engineered to interact with one another in a controlled manner, enabling the entire system to perform computations. When arranged in specific patterns, these cubes can represent and manipulate data, effectively functioning as a mechanical processor. This approach eliminates the need for traditional electronic components, which can be bulky, energy-intensive, and susceptible to heat and electromagnetic interference.
How It Works: The Mechanics of Data Storage
The operation of the kirigami-inspired mechanical computer relies on the precise manipulation of the polymer cubes. Each cube can exist in multiple states, depending on its configuration and the forces applied to it. These states correspond to binary data, with different arrangements representing different bits of information.
To perform computations, the system applies external forces to the cubes, causing them to reconfigure. This process is analogous to the way electronic circuits change states to process information. However, instead of relying on electrical signals, the mechanical computer uses physical interactions to achieve the same result.
The polymer cubes are designed to be highly responsive, allowing for rapid and precise changes in configuration. This responsiveness is crucial for performing complex computations in a timely manner. Additionally, the cubes can be reset to their original state, making them reusable for multiple operations.
Potential Applications and Impact
The development of kirigami-inspired mechanical computing systems opens up exciting possibilities across various fields. Here are some potential applications and impacts of this technology:
-
Energy-Efficient Computing: Mechanical computers have the potential to operate with significantly lower energy consumption compared to electronic systems. This makes them ideal for applications where power efficiency is critical, such as remote sensing and environmental monitoring.
-
Harsh Environments: Electronic components are vulnerable to extreme conditions, including high temperatures, radiation, and electromagnetic interference. Mechanical systems, on the other hand, can be more robust and reliable in such environments, making them suitable for space exploration, deep-sea missions, and industrial applications.
-
Medical Devices: The biocompatibility and flexibility of the polymer cubes make them promising candidates for use in medical devices. They could be used to develop implantable systems for monitoring and treating health conditions, offering a safer and more durable alternative to electronic implants.
-
Education and Research: Mechanical computing systems provide a tangible and intuitive way to understand and demonstrate computational principles. They can be used as educational tools to teach concepts of data storage and processing, as well as in research to explore new computational paradigms.
-
Sustainable Technology: With growing concerns about electronic waste and the environmental impact of traditional computing devices, mechanical systems offer a more sustainable alternative. The use of recyclable materials and the potential for lower energy consumption make them an attractive option for environmentally conscious technology development.
Challenges and Future Directions
While kirigami-inspired mechanical computing presents numerous advantages, it also faces several challenges that need to be addressed for widespread adoption. Some of the key challenges and future directions for research include:
-
Scalability: One of the main challenges is scaling up the system to handle larger and more complex computations. Researchers need to develop methods to integrate more polymer cubes into a single system while maintaining precise control over their interactions.
-
Speed and Efficiency: Mechanical systems generally operate slower than electronic ones. Enhancing the speed and efficiency of mechanical computations is crucial to make them competitive with traditional computing technologies.
-
Durability and Longevity: The mechanical components must be durable enough to withstand repeated use and environmental stresses. Improving the materials and design to ensure long-lasting performance is essential for practical applications.
-
Integration with Existing Technologies: To maximize their impact, mechanical computers should be able to integrate with existing electronic systems. Developing hybrid systems that combine the strengths of both mechanical and electronic components could lead to more versatile and powerful computing solutions.
-
Cost-Effectiveness: Reducing the cost of producing and maintaining mechanical computing systems is necessary for their commercial viability. Advances in materials science and manufacturing techniques will play a crucial role in making these systems affordable and accessible.
Conclusion: A Promising Future for Mechanical Computing
The development of kirigami-inspired mechanical computing systems represents a significant leap forward in the field of computational technology. By harnessing the principles of kirigami and the unique properties of polymer cubes, researchers have created a novel approach to data storage and processing that bypasses traditional electronics.
This innovative technology holds immense potential across a wide range of applications, from energy-efficient computing and medical devices to education and sustainable technology. As researchers continue to address the challenges and refine the design, kirigami-inspired mechanical computers could revolutionize the way we think about and use computing systems.
The future of mechanical computing is bright, with the promise of new possibilities and breakthroughs that could transform industries and improve lives. As this technology evolves, it will be fascinating to see how it reshapes our understanding of computation and opens up new frontiers in science and technology.